Structures are designed for specific purposes. We say that form follows function.
Types of Structures
Solid Structures
Solid structures are solid through and through even though it may have some gaps and small holes in it. The majority of its structure is solid. A dam for example may have service tunnels and electrical lines running through it, but is otherwise solid. Solid structures have the advantage of being simple in design and generally do not require as much training to build (except for the use of special building materials). They are, however, heavier and use more material compared to other types of structures.
Examples are dams, main support colums, foundations etc… .
Shell Structure
Shell structures are mostly hollow, but have a solid outer skin. Their primary use is as containers and for protection (often both). Shell structures can be remarkably strong and use way less material than solid structures. However, they require more knowledge to build. Examples are:
Domes, bowls, barrels, our skull, nuts and eggs.
Frame Structure
Frame structures are made of many individual parts that are connected to each other in complex ways. The key to strong and useful frame structures is the specific way that parts are attached and the manner in which they are attached. Frame structures are more flexible than other comparable structures made from the same material. They usually require greater knowledge and skill to assemble, causing greater construction cost, but use significantly less material. They are the most sophisticated of structures.
Examples are the framing of houses and buildings, our skeleton, spiderwebs and the internal structure of bones.
Combination Structures
Many structures are combinations of two or all three types of structures. A house, for example, has a solid foundation, frame walls and roof trusses and is covered by flat sheets of wood and drywall, making it a large shell structure.
Forces
A force is a push or a pull. Forces act on all structures, big or small, and the structure must be able to withstand the forces. Structures experience both internal as well as external forces. External forces act from the outside, like wind blowing on a house wall, while internal forces act from the inside of the structure, like the tension in a tendon or ligament that holds a joint together.
Describing Forces
Many quantities in nature/science have just one “dimension”. They are basically a count in a certain quantity and that’s it. For example, I could try to find out what the length of a piece of wood is. The answer is a simple number, like 24 cm. Such quantities are called scalar quantities. They are fully described by a magnitude (size) of the number alone.
Forces, on the other hand, have both a magnitude and a directionality. A force pushing left will have a dramatically different effect to one pushing right, even if they have the very same strength (magnitude). The direction in which the force is acting is an essential part in fully describing it. Such quantities are called vectors and are represented with an arrow. In terms of what a force will do to a structure, there is even more to a force. It’s not just how strong a force is and in which direction it is acting, but also where on the object it is acting. We call this the Point of Application. Structures often have stronger and weaker points and a force acting on a weak spot can have devastating effects.
When we draw forces we can use arrows to express all three aspects of a force: Magnitude, Direction and Point of Application.
Magnitude:
The magnitude of a force describes its strength and is represented by the length of an arrow. Most unfortunately, magnitude is often represented by the thickness of an arrow in drawings even in the odd textbook. This is really bad practice and you should not adopt it.
Direction:
The direction of a force describes the direction in which it is acting and is represented by the direction of the arrow ( I’m almost embarrassed to write this, but it is that obvious).
Point/Plane of Application:
The point of application of a force is where it is applied on a structure. This is a third aspect of a force that applies when we consider the effect of forces on structures. Consider a lever, for example. Where we push on a lever determines the mechanical advantage the lever will produce for us. We instinctively will slide back on a lever if we find it too hard to use it. That changes the point of application while keeping everything else the same. The point of application of a force is expressed by where the arrow attaches to the structure (tip or origin).
Try to work out in which way the two forces are the same and different in the following drawing:
Magnitude:
Direction:
Point of Application:
Loads
Structures need to be able to support loads. Loads are caused by forces acting on the structure.
The total load on a structure is the sum of its static and dynamic load. The static load is the load due gravity on the structure itself. Consider that many strong building materials are also very heavy, which adds to the static load on a structure before we have even begun to load it up with anything useful.
Building a structure from the strongest material that is available can be a bad idea, because the material may be very expensive, rare or too heavy for the building you’re trying to build. One famous example of a structure made from material that is strong but too heavy for its location is the Leaning Tower of Pisa. It is built from beautiful white marble, which is very heavy. Too heavy for the soil it was place on and concequently, it started to shift the soil underneath its foundation and started to lean.
The dynamic load is the load due to forces that change while they are acting on the structure. Consider a truck driving across a bridge. It constantly changes its point of application on the bridge. The force due to wind change magnitude, direction and point of application with changes in the wind pattern.
Internal Forces/Stress:
Loads produce internal force or stress within a structure and we must design them with that in mind.
Compression:
Compression occurs when two forces push towards one another (squeezing). The object may respond by squeezing together or buckling. Solid structures tend to be used the most if compression is expected (example: a house foundation is made of poured concrete).
Tension:
Tension occurs when two forces pull away from one another (stretching). The object may respond by stretching or ripping. Depending on the circumstances we may use elastic materials or materials with high tensile strength like steel cables. Some materials that are great at resisting compression are terrible at resisting tension. Concrete, for example, is great for compression but must be reinforced with steel bars if any degree of tension is expected.
Torsion:
Torsion is a stress that results from a twisting motion. In nature, torsion can be extremely destructive. Structures that need to resist torsion are often designed with some level of built in flexibility. Frame structures, for example, have such a built in flexibility, stemming from a small amount of mobility in each of its many joints.
Shear:
Shear is a stress that occurs when two forces just slide past one another. The structure rips, or is cut, right along the line where the two forces slide past one another. We make use of this in just about every cutting tool, especially scissors. Resisting shear tends to be a simple question of structural strength. You either have it, or you don’t.
Preventing Failure:
Every day, we depend on structures doing their job. cars and buses bring us safely to where we want to go, bridges and houses stay strong, carrying their burden. Sometimes, though, we witness the failure of such structures, often with tragic consequences.
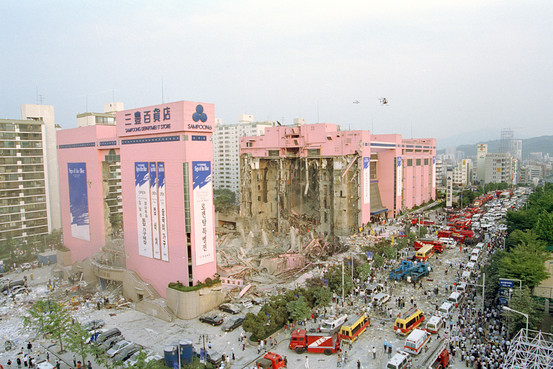
Designers try to anticipate the intensity and duration of use, the exposure to factors like heating and cooling that might fatigue materials, and the human factor, which often means that people will abuse and misuse objects.
While a designer can’t and shouldn’t be held accountable for the poor judgement of other people, it is still a good idea to build in a safety cushion to account to lapses in common sense. Such extra capacity in ability to support loads, for example, is called a “margin of safety”. Consider that if an elevator fails catastrophically, the human cost may be very high. The inside of elevators list the maximum load that should be in the cabin, but who’s going to calculate the total load, much less ask for the individual weight of passengers, before getting on. It’s much better to make the actual ability of the elevator higher than advertised.
Another way to increase the safety of structures is to add sensors to it that monitor crucial values. An elevator, for example, might have an overload sensor that disables the cabin from moving if a critical load value is surpassed, houses are now required to have functioning carbon monoxide and smoke detectors, etc…
Strength and Stability:
Strength:
As far as structural strength is concerned, there is, of course, the material’s innate strength. Some materials are simply stronger than others simply based on the very nature of the material. Steel is simply stronger than a piece of pine, but a beam made of wood is lighter than a steel beam. In order to maximize strength while keeping weight to a minimum we can employ a number of tricks:
- Triangles, Curves, Domes
Using triangle shapes, curves and domes we can distribute the load over more than one location and lessen the impact. -
Trusses are extremely common in building and bridge construction because they provide significant reinforcement with little material. Notice how they are basically a collection of triangles. - I-beams:
I beams are often used as main support beams in house and building construction. The have a strength that compares to that of a solid steel beam of the same size, but uses only a fraction of the material. - Corrugation
- Lamination
Notice how the direction of the wood is switched in each layer. Wood breaks most easily along the direction of its grain. We could consider wood to have a directional weakness. By gluing each layer with the grain facing a different way by 90 degrees, we can eliminate this directional weakness. A piece of plywood is much stronger than a piece of solid wood of the same kind and size.
Stability:
Stability measures how much it takes to make a structure fall over. A structure will fall over as soon as we bring its centre of gravity outside of its support base (assuming it’s not anchored or tied down etc…).
Wide support bases and less height both bring the centre of gravity lower ot the ground, which makes it harder to bring outside the support base by tipping.
Lifespan of a Product
Every product has a lifespan. When a manufacturer designs a product, they have to answer the question of how long it should last before it wears out. If it wears out too quickly, consumers will feel like they’re not getting their money’s worth. If it lasted a very long time, either the manufacturer wouldn’t make a profit or the item would cost too much because longer life span usually comes with using superior, and thus more expensive, raw materials and manufacturing methods. When a manufacturer deliberately designs a product with a limited life span, it is called planned obsolescence. Finding the right length of time before a product fails is a delicate balance between the desires of consumers (long life and low purchase cost) and those of the manufacture (short life span and high purchase cost).
When a product has reached the end of its life cycle, it needs to be disposed of. Often enough the entire item just ends up in our landfill ad that pollutes our environment and is wasteful. Designing a consumer item should also keep in mind how much of an item can be recycled and reclaimed and how easy it is to do that.